3-Dimensional Screen Printing of Pharmaceuticals
- Frances Hoggard
- Feb 3, 2023
- 5 min read
Updated: May 22, 2024
How Does It Work and How Can It Benefit Patients and Healthcare Providers?
When you first hear about the concept of 3D printing of medication, you might wonder how exactly the manufacturing process works. Does it produce medications comparable to conventionally manufactured drugs in terms of drug delivery, durability, and safety? What are the advantages of using 3D printing pharmaceuticals? How can this technological advancement ultimately benefit patients and providers more than conventional medications?
How Does 3D Printing of Drugs Work?
Gathering the Materials
The first step in 3D pharmaceutical printing is gathering the necessary materials to create a semi-solid paste. This paste contains:
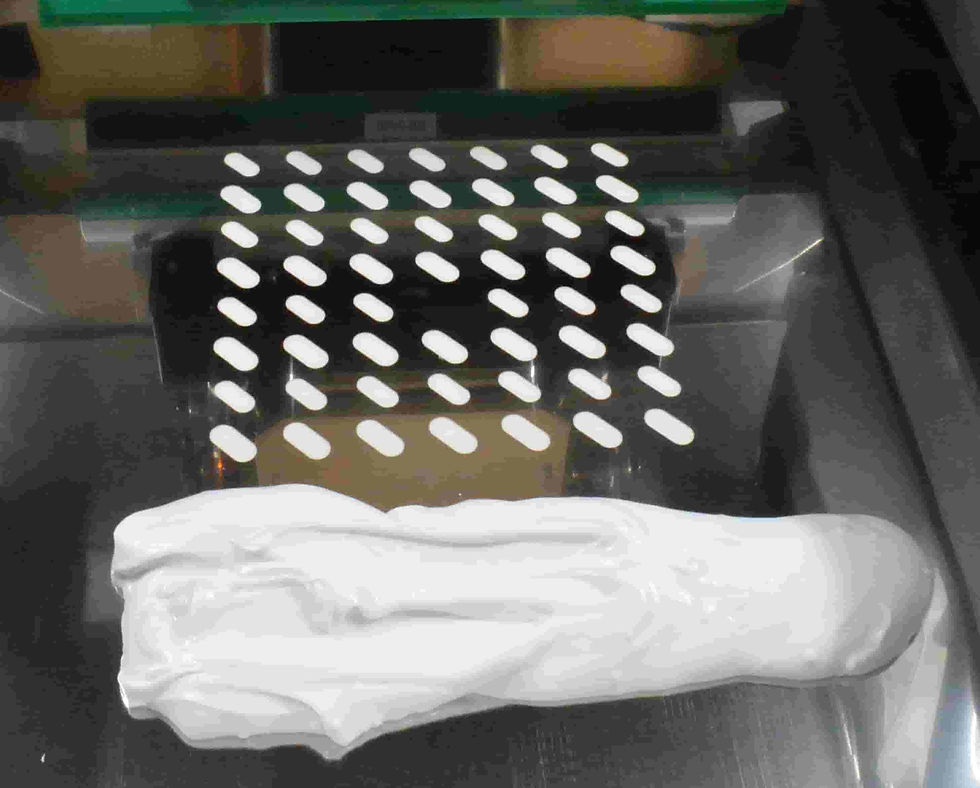
the drug’s active pharmaceutical ingredient (API)
modifiable polymers such as polyvinylpyrrolidone, crospovidone, microcrystalline cellulose, or hydroxypropyl cellulose
water
starch
glycerol, and
sunflower oil.
The various properties of the polymers, which can be altered according to desired effects, allow for manipulation of the timing of API release (immediate vs. delayed vs. extended release).
Making the Paste for the Tablets
The next step is making the paste used during the printing process to create the tablets. The API is ground up into a fine powder. Amounts can be easily altered to change the formulated dose within each tablet. Each ingredient is gradually added into a mixture at specific intervals and in exact amounts while constantly being stirred under room temperature conditions.
Inputting Printer Parameters
The third step involves setting up the 3D printer parameters, including the:
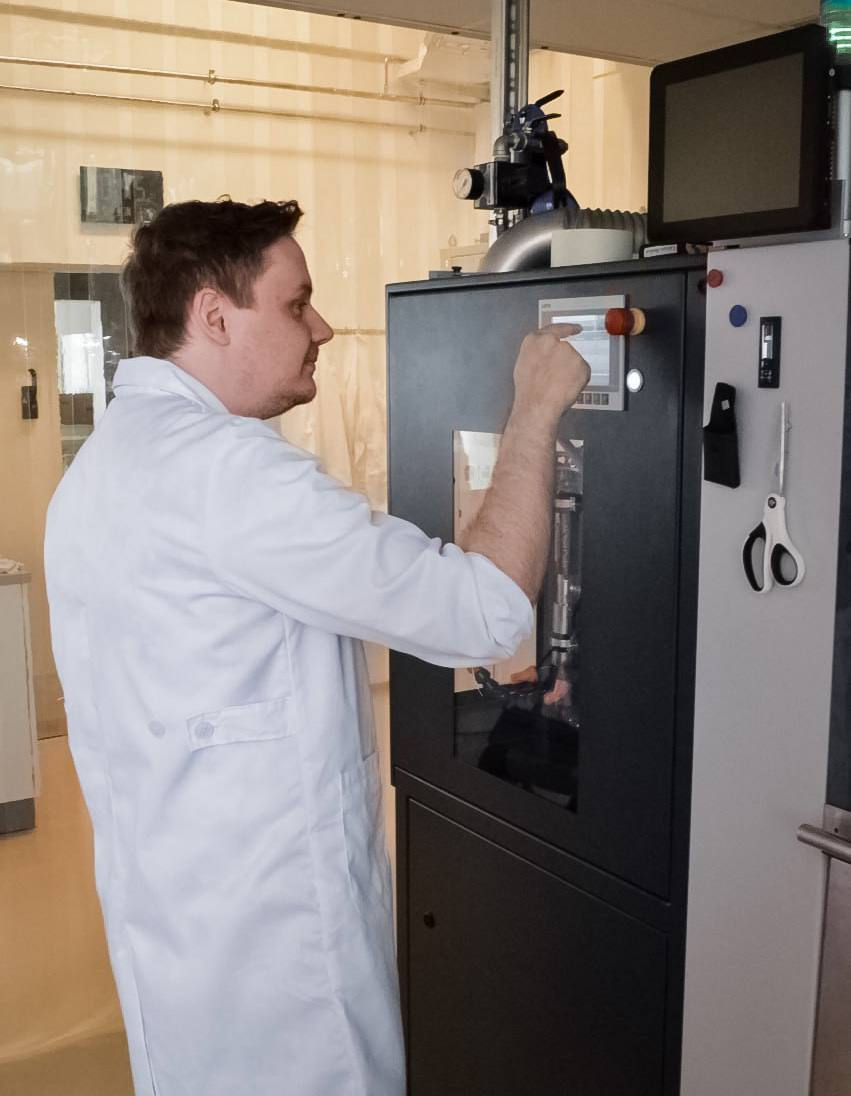
flooding and printing squeegee speeds (mm/sec),
off-contact distance between the printer screen and the substrate plate (mm),
height increment for screen elevation depending on the thickness of each printed layer (µm),
dryer temperature (degrees Celsius),
dryer time (seconds) to ensure solidification of each printed layer prior to the application of a new layer on top, and
the number of printed layers to achieve the desired tablet height.
Additionally, specifications for tablet geometry and dimensions, including width and length for cuboid, oval, and grid shapes, and diameter for disk and donut shapes, must be programmed into the printer. Tablet shape determines the surface-area-to-volume ratio of the tablet, a critical component for determining the rate of API release to the body.
Printing the Tablets
The manufacturing process repeats printing cycles based on these predefined parameters and involves a series of steps per cycle.
The printing screen is positioned over the substrate plate from a predetermined distance.
The flooding squeegee fills the openings in a screen mesh with the formulated paste.
A reverse printing stroke brings the paste and the mesh into contact with each other, transferring the paste onto the substrate panel, while the printer screen returns to its original position.
Prior to initiating printing of the next layer, the substrate plate with the recently printed layer is transported to an external dryer where paste solvents are removed by convention using dry, heated air. This solidifies the printed layer before returning to the printing station to add the next layer directly over top of the existing layer.
After the printing screen is aligned exactly with the bottom layer and elevated by the predetermined thickness of the previously printed layer, the cycle repeats until the desired tablet height is achieved.
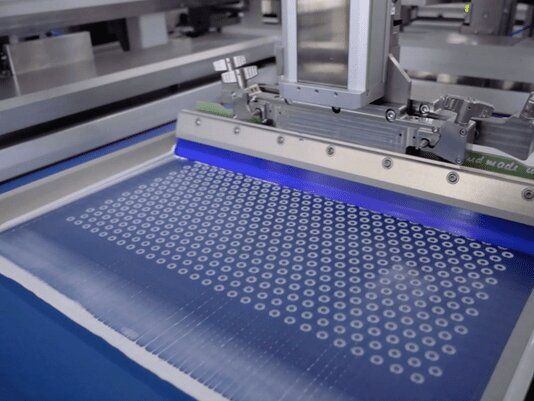
For an added layer of patient protection, Quick Response (QR) codes can either be printed directly on top of the tablets with Inkjet printing techniques or be embedded into the tablet itself.
These QR codes can contain a wealth of information, including patient name, manufacturer ID, expiration date, possible drug interactions, batch number, administration route, and anti-counterfeiting measures, such as holograms or fluorescent inks to prevent falsification of QR codes.
Do 3D Printed Drugs Compare to Conventionally Manufactured Drugs?
Researchers subjected the 3D-printed drugs to a series of tests to confirm mass uniformity, hardness, friability, dissolution in an acidic solution with a pH of 1.2 (designed to simulate the gastric environment), and drug content within each tablet. The drugs demonstrated:
acceptable differences in lateral tablet size (3%) and vertical height (less than 6%) compared with projected sizes (which allows for modifications by adding water content and changing drying duration),
high resistance to crushing and breaking,
low weight loss (less than 0.1%) due to friability,
adherence of 3D printed pharmaceuticals to the general rules governing the release of solid oral dosage forms based on surface-area-to-volume ratio,
high uniformity (99.5% ± 2.1%) compared with projected drug amounts contained within each tablet regardless of size or shape.
These results confirm the capacity of the 3D screen printing process to mass produce uniform drugs that effectively deliver expected drug dosages within accepted parameters required of conventionally manufactured pharmaceuticals. These 3D-printed pharmaceuticals would still be required to undergo the same rigorous clinical trial testing for regulatory approval as conventionally manufactured drugs to ensure drug efficacy and patient safety.
How Can 3D Printing Benefit Patients and Providers?
The nature of 3D printing through using additive manufacturing benefits patients and providers alike. Additive manufacturing of these tablets in layers allows for the following:
the flexibility of the design of the drug delivery system in terms of shape, size, API content, and drug release characteristics,
customization of medication prescriptions catered to meet the unique needs of each patient depending on their individualized disease activity state and rates of drug metabolism as well as ease of administration and aspects of disease management,
mass production of more complex drug delivery systems, such as combination therapies, to reduce polypharmacy and improve patient adherence to prescribed medications by treating multiple comorbidities using a single tablet,
quicker exploration and formulation of drugs during the clinical trial process to determine optimal dose parameters for efficacy and safety,
prevention of drug counterfeiting down to single tablets using printed in-drug QR codes,
reduction of medication errors during dispensation and dosing in pharmacies and hospital settings with QR codes,
remote monitoring of patient adherence requiring QR code scans prior to dose administration, and
reduction of drug interactions (especially with over-the-counter supplements), and rapid digital communication of the most up-to-date medication information enabled by on-demand data access through QR codes.
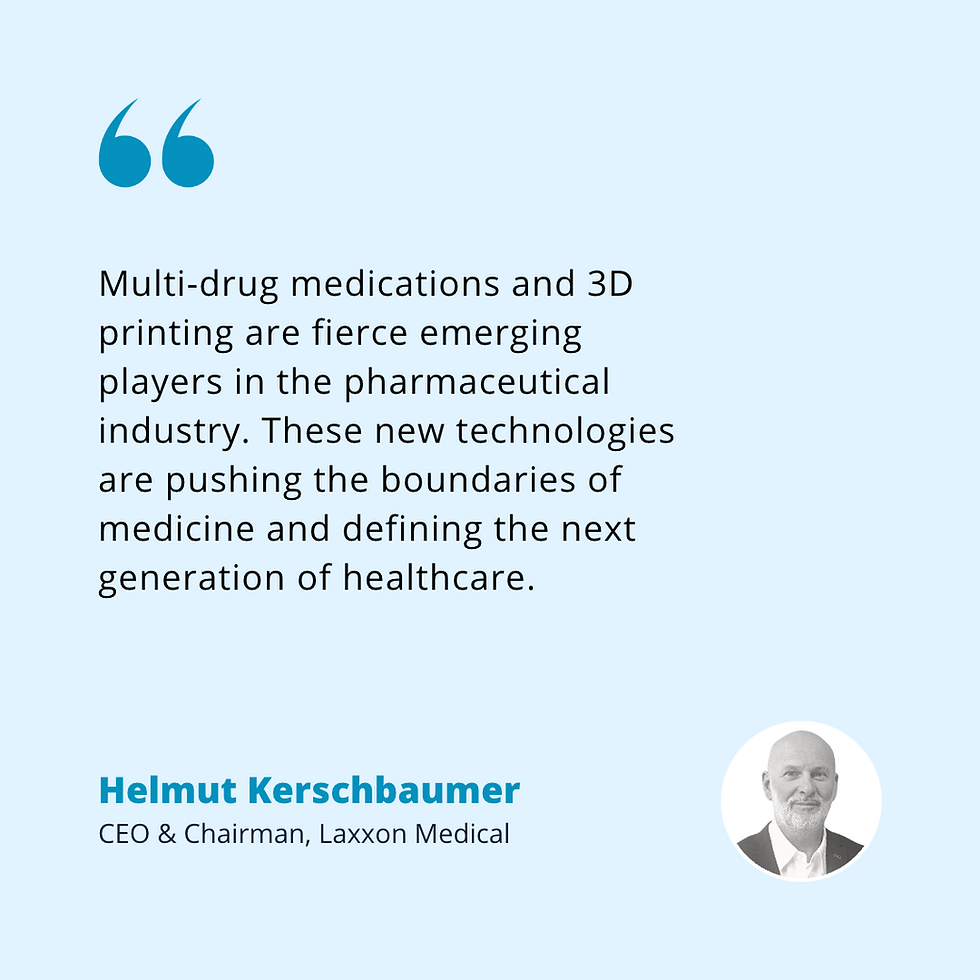
Scanning QR codes on tablets before consumption may prevent mistaken overdoses of the same medication when patients combine pills of similar shapes, colors, and sizes in weekly or daily pill boxes.
Conclusion
There are potential limitations with the universal application of QR codes since not everyone can afford, access, or know how to use smartphones or tablets.
Nevertheless, 3D screen printing of pharmaceuticals opens the doors to the mass production of easily customizable, precision medications while upholding — and possibly even enhancing — patient safety, drug efficacy, and optimized disease management.

Laxxon Medical is dedicated to engineering patented 3D pharmaceutical solutions which optimize products and benefit patients. Our goal is to establish SPID®-Technology as a manufacturing process that has the individual and the pharmaceutical partner in mind.
To keep up to date with SPID®-Technology and Laxxon Medical news and announcements, follow us on Twitter and LinkedIn.